Hydrogen: The Water-Stuff Of To-day, Yesterday, and To-Morrow
There’s a lot of talk these days about hydrogen. After all, it is the most abundant element (something like 90% of all of the non-dark-matter in the universe). It burns clean and green, producing only water! Cool stuff, right? So, as we’re thinking about decarbonization, is there any possibility that we could replace fossil gas (methane, mostly) with hydrogen? The short answer? Well, not really. Longer answer? It’s possible, but, as in the case of ammonia replacing hydrochlorofluorocarbon refrigerants and everything else I write about on this blog: it’s kinda possible, but… it’s complicated. It’s also more complicated than many of the toxic positivity boosters on LinkedIn would have you believe.
Hydrocarbons, as we’re aware, usually come from deep underground. Their combustion generates planet-warming carbon dioxide (plus usually other nasty particulates). Hydrogen, in contrast, one of the primary atomic constituents of hydrocarbons, doesn’t fit neatly into any box, literally or figuratively. It comprises much of the mass of our sun, for example. It exists in outer space. And, here on earth, it can be purified from water, which is one of the most abundant substances on the earth, and much easier to obtain in large quantities than, say, uranium (which must then be purified and enriched in a complex process before being used to create a fission reaction, then creating steam and finally electricity), coal (which must be mined, processed, and then burned to create steam in the same type of process), or, certainly, lithium and other rare earth metals powering The Great Batteries of To-Morrow for the 9,000-lb. SUVs that, Gretchen Whitmer claims, will decarbonize transportation.
At face value, it sounds like a slam dunk, right?
Maybe! Hydrogen has a lot of potential in fuel cells and even combustion heating, especially given the relative ease of refining it compared to things like lithium or cobalt. But in thinking about the potential industrial future of hydrogen, it might be valuable for us to explore a bit about its past– to understand how it’s an element that we know a lot about but have never really figured out in its entirety.
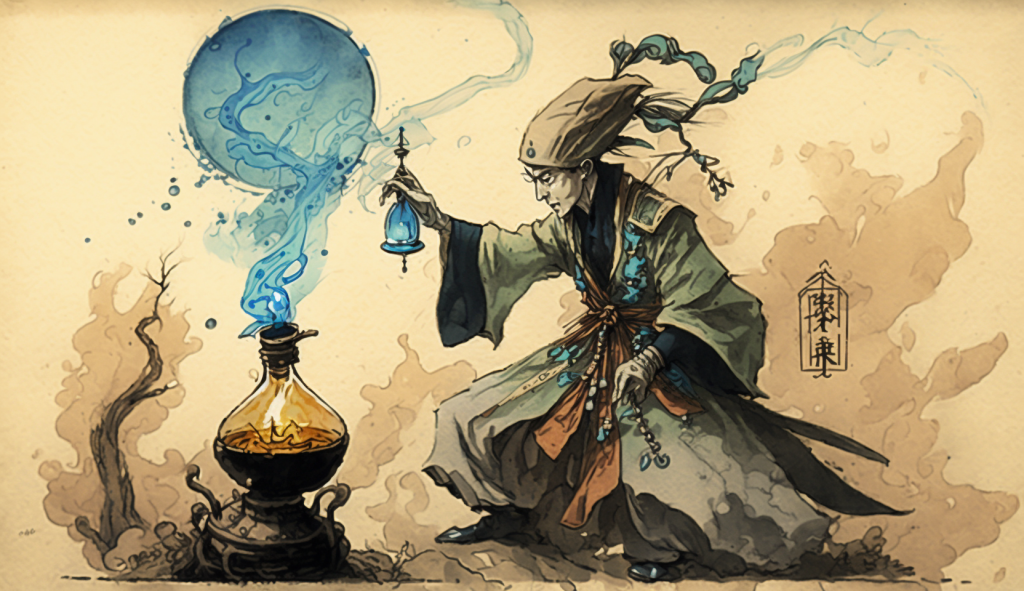
Hydrogen Through The Ages
Today isn’t the first time in history society has been smitten with this craze over this tiny atom. But it’s blown up– no pun intended- in recent years with the growing growing recognition that we should probably figure out a way to mitigate this whole climate change thing before we all die. I was thinking about this most recently after watching Glass Onion, in which a proprietary, hydrogen-based substance plays an important part in one plot thread. It’s a subject that energy experts seem to dance around a bit, because “hydrogen as simple solution to the carbon crisis” is one of those, “Napoleon could conquer England if he had a bridge” kind of things. Even if one were able to figure out how to refine hydrogen cost-effectively, say, from water, at a huge, industrial scale, it would still have to deal with the challenges of the element’s extreme flammability, not to mention what is probably a bigger challenge– of storing and distributing it.
Distributing it, then, is not just a matter of networks of pipelines, trucks, or trains, but also of the technology used in the process, because hydrogen creates some unique challenges at the atomic level (more on this later). We’ve made a lot of progress, and I’ve gone from thoroughly skeptical to cautiously curious.

A Brief History Of Phlogiston, Whatever That Is
The flammability of a gas that had some relationship with water was recognized as early as the 17th century, when super smart scientists– who had just in the preceding couple of centuries gotten over that minor operational hurdle of being threatened with being burned at the stake for suggesting the earth was round- guessed that living things were full of some sort of chemical that could burn very easily. They knew that water was involved, but they weren’t sure how. Early experiments found that you could produce a highly flammable gas by mixing metal shavings with various solutions.
So important was the burny element here that a number of scientists– variously, Johann Joachim Becher (1635-1682), Georg Ernst Stahl (1659-1734), Robert Boyle (1627-1691), Henry Cavendish (1731-1810), and Joseph Priestley (1733-1804)- developed an entire body of “kinda sorta true, but missing the actual point” theories around what they ended up calling phlogiston (“burny stuff” from Greek). Priestley was the last of the phlogistonians, holding out his beliefs even after newer scientific discourses had emerged to basically prove him wrong.
Phlogiston was based in the primitive scientific notion of old that everything is made up of four elements: air, water, fire, or earth. I say it’s “kinda sorta true” because if people are mostly water and water is substantially made of hydrogen, you can indeed argue that we are all made up of burny stuff, given that hydrogen burns and is a primary constituent of water. Notably, this was all way before petroleum, whose discovery in the 19th century would transform our understanding of energy, energy distribution, and resource extraction. This point will also be important later because of how much hydrogen is derived from petroleum and fossil gas.
It’s interesting that the scientists who figured out how to isolate this burny compound didn’t really make the connection between lift. Nay, that came later, from the French! And this is where the first broad hydrogen application arose. Interestingly, Jules Verne may well have been the first to have proposed the notion of water as the new fuel source of the future as early as 1874 in The Mysterious Island, a novel in the same vein as The Swiss Family Robinson, Robinson Crusoe, and other absurd adventure novels from the 18th and 19th centuries. In L’ÃŽle Mysterieuse, Verne’s character explains:
“I believe that water will one day be employed as a fuel, that hydrogen and oxygen which constitute it, used singly or together, will furnish an inexhaustible source of heat and light, of an intensity of which coal is not capable.”
Cyrus Harding, character in Jules Verne’s 1874 novel, The Mysterious Island
By this time, scientists knew that hydrogen was its own thing, and phlogistonian theory had been dismantled completely in the preceding century. But it would take decades for hydrogen to become industrially or commercially popular.
Â
Remembering The Hindenburg, And Those Who Preceded Her
Many decades before the lights of the Goodyear Blimp read, “Ice Cube’s A Pimp,” dirigibles, airships, zeppelins, and whatever you want to call them, were given their lift by hydrogen rather than helium, which now fills balloons and the few dirigibles we do still see in our society. The airship itself came out of the recognition that you could create lift by having something that was lighter than air, and this recognition ironically developed somewhat separate from the discovery of the hydrogen that would later provide that vital lift. The Italian Jesuit priest Francesco Lana de Terzi figured this out in the 17th century with plans for an airship that was lifted by spheres that contained, well, nothing— a vacuum. To this day, such a design remains physically impossible to build, because the outside atmospheric pressure would crush anything that was light enough for the vacuum to constitute a meaningful, well, lack of mass.
A crushed orb, of course, does not make for a great lift mechanism.
But Frankie inspired others, and preliminary designs for balloons whose lift was provided by hot air were developed as early as the early 18th century. Perhaps an example of simultaneous invention or multiple discovery, roughly the scientific equivalent of convergent evolution, the first three proper flights in a hot air balloon– the first powered by heated air, the second powered by hydrogen, and the third a manned flight in a hydrogen balloon– all occurred in 1783 in France. But it took another century to produce a commercially viable transportation medium using this new medium of buoyancy, because of the challenge of producing anything ranging from a lightweight balloon frame to a large amount of pure hydrogen.
Discovery vs. Scale
The lag here was in part because a lot of the science that was necessary for producing airships was pretty much completely unrelated to the science required to refine large amounts of hydrogen necessary to provide buoyancy to a giant object. While fluid dynamics had been understood in some fashion for thousands of years– think sailing, or Archimedes’ bathtub- the concept of aerodynamics was mostly brand-spanking new in the 18th century, and scientific understanding was relatively limited until the latter half of the 19th century.
Maneuverability was a key challenge for the development of the balloon. We can recall that trains were pretty new in the 19th century, while propellers were an 18th century invention (similar in design but ultimately only a distant cousin to the older windmill, a millennia-old technology). Propellers took another century before the technology was refined enough to develop things like giant steamships or zeppelins with the ability to actually move around. Accurate but primitive science required precision in measurement, but the development of things like precision gears or propellers required much more advanced manufacturing technology.
It’s also important to remember that there’s a big difference between discovering that something will work on a limited, one-off basis, and figuring out how to scale that technology to be able to build a thing that multiple people can use in an industrial or commercial context. This lesson is as important when thinking back to 1783 as it is when thinking about the modern day. It’s the same logic behind why it’s possible to develop a functional prototype for an electric car but thoroughly difficult to develop a company that can profitably mass produce durable, comfortable, and safe electric cars. Today, we have other challenges, like the fact that our obsession with scarcity means that everyone is trying to make as much money as possible at all times, and higher prices may hinder market adoption for new and experimental machines or products.
The Age of Industrial Refinement
The age of the hydrogen airship arrived in a spectacle of industrial innovation and wonder appropriate for fin-de-siecle Europe when Count Ferdinand von Zeppelin launched his test fight over Lake Constance. But while this birthed a new age of air travel preceding the development of much faster commercial airliners, it would take decades for scientists to figure out how to more efficiently refine hydrogen.
Gazing not skyward but groundward, Fritz Haber and Carl Bosch developed a method for refining ammonia at industrial scale. Ammonia was used principally in fertilizer and in bombs, so, somewhat different from the idea of building a stately pleasure craft to sail through the clouds, but still reliant upon the same buoyant medium of hydrogen. While 19th and 18th century scientific experiments were limited in scale by the available technology if not the limited understanding of chemistry, the 20th century offered industrial innovations such as the ability to produce large, steel vessels that could be pressurized and heated relatively easily in comparison to the methods of old. Messrs Haber and Bosch figured this out beginning in the 1910s, going from producing a few ounces of ammonia in a day to producing tons per day by 1914. Hydrogen in these days was produced by a simple chemical reaction to heat coal and water to produce carbon monoxide and hydrogen (C+H2​O→CO+H2). Electrolysis was a known solution, but electricity was rare and expensive in these days.​

Steam methane reforming (SMR) emerged concurrent with the discovery of all of the wondrous things that can be done with petroleum and its related substances. Through heat and pressure, many things are possible, and this is where the Haber-Bosch process comes in, too– the ability to produce a lot of ammonia from nitrogen and hydrogen heated at high pressure. Of course, the production of steam requires a lot of energy, and the production of hydrogen through SMR is profoundly un-green. Characteristically in terms of the 20th century’s affinity for burning a lot of fossil fuels, SMR became the dominant method of hydrogen production after the development of the Haber-Bosch process.
Fossil gas (a.k.a. “natural” gas) contains methane and often a number of other gases in smaller amounts. Ethane, for example, is often used in the production of plastics. Another important constituent of fossil gas is helium, which became far more popular after a certain, notorious airship blew up in 1937. The Hindenburg disaster was unfortunate for the future of wasserstoff, since hydrogen came to be synonymous with “airship blowing up over New Jersey.”
Â
Ok, Cool. What about helium? What’s Going On There?
In terms of its industrial use as a medium for buoyancy, helium can be thought of as hydrogen’s educated cousin. It’s more stable, doesn’t blow up, and is a noble gas. Helium, in comparison, was only isolated centuries after hydrogen was first discovered– even if ‘discovery’ in days of yore just meant some dude in a frilly dress going, “Zounds, your Grace, what think you is this burninge water-stoffe?!” at the time. It was named after our great celestial progenitor (“helios,” a.k.a. “the sun”) because of the sunshiney yellow lines that showed up when a few scientists were looking at the sun to try and figure out what it was made of (this is how a lot of astrophysics works, as it turns out– looking at celestial bodies through special instruments and guessing what they’re probably made out of based on the color lines that show up). This was in the 1860s, and it wasn’t until around the turn of the century that scientists would figure that helium can actually be refined from certain rare rocks (a concept I was personally unfamiliar with until this futurist rapper cornered me in downtown Detroit several years ago to tell me about how the moon is going to be mined for helium).
Like hydrogen, helium can also be refined from fossil gas, because it is often present in small quantities (ranging from a fraction of a percent to several percent). Gas is cooled to a low temperature, and the helium is easy to separate because helium has a much lower boiling point. Helium grew in popularity for balloon purposes during the first World War, paralleling the broad development of petro-gas extraction and distribution systems.
It wasn’t until the aftermath of the Hindenburg Disaster in 1937 that the dominant paradigm began to shift. Given the recognition that hydrogen was so flammable, why not switch to a buoyancy medium (is there a specific term for this? A Buoyande?) that didn’t threaten to kill everyone if something minor went awry?
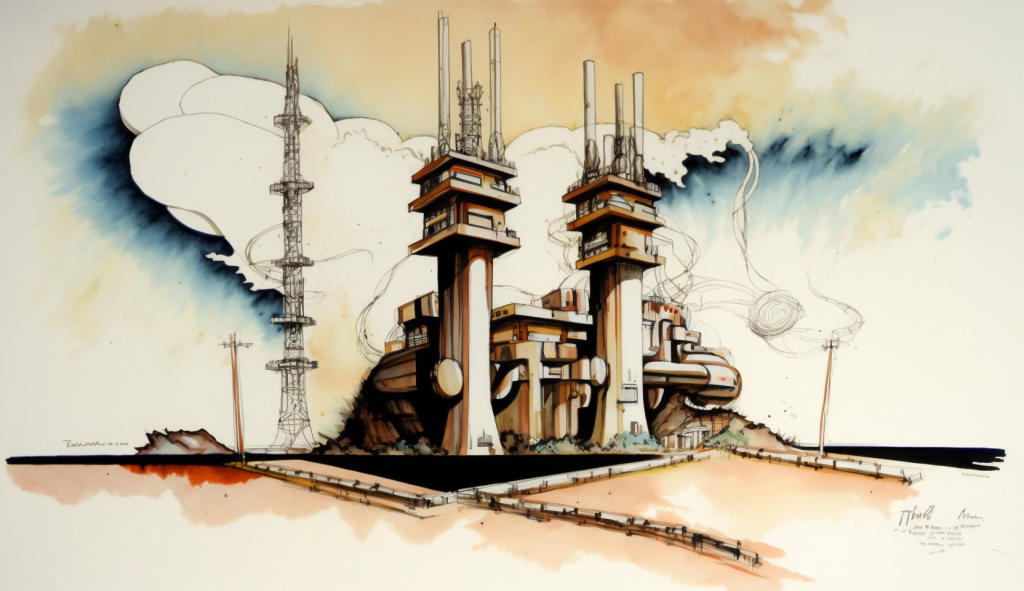
“Too Good To Be True” vs. Basic Molecular Physics
In spite of the fact that it is quite literally everywhere, hydrogen does pose some challenges at an atomic level if we’re thinking about delivering it inside our houses. Unlike helium, hydrogen is not a noble gas, which means that it is going to be more reactive. Hydrogen’s lower energy density also means that it would require more pressure (and probably a lower temperature as well) to transport. Both of these combine to be problematic in the design of systems. But methane and propane are also compressed, and compression in distribution systems is well-understood since we’ve been doing it for a couple of centuries now, beginning with the emergence of municipal gas street lighting (and subsequently gas lighting in residences and buildings) in the 19th century.
The bigger problem is that hydrogen can infiltrate the crystal lattice of some metals, making them more brittle and therefore susceptible to cracking. This “fuel vs. medium through which the fuel is transported” challenge is hardly unique to hydrogen. It’s kind of like how proposals to phase out hydrocarbon refrigerants (a.k.a. most refrigerants that are in use today) in favor of ammonia, which is less environmentally problematic, but, importantly, can’t be used in copper because of Science. Most cooling coils are copper, meaning that replacing all of them across a whole society would cost, you know, thousands of dollars per dwelling unit, etc., so imagining a transition to a far greener refrigerant requires the ability to imagine replacing effectively every last refrigeration unit in use in residential applications in the entire country and most commercial and industrial ones, too.

Managing the distribution process for pressurized hydrogen is complicated, as it requires comprehensive attention to engineering and design, using certain alloys of metals that are less prone to the infiltration by hydrogen. Creating a safe, stable distribution network, though, is a question, as it’s unclear that fossil gas distribution networks could simply be retrofitted to handle higher-pressure hydrogen distribution. Compressed and liquid hydrogen have a respectable energy density compared to alternatives, if you’re able to get past the enormously energy-intensive process not only of refining it, but also cooling it (for liquid).
The bigger problem, though, and especially relevant in terms of discussing the credibility of hydrogen as a fuel to help us on the path toward decarbonization, is that we’re still stuck on the SMR process of hydrogen production. Around half of global hydrogen come is “blue” hydrogen, which means that it comes from fossil gas, while only single percentage points come from electrolysis and “greener” methods. This isn’t to say that this isn’t changing with the advent of broadly available (and affordable) renewable energy, but it does call into question the vapid boosterism of the pro-hydrogen folks, who seem all too keen to gloss over the fossil fuel foundations of this ubiquitous, but still surprisingly poorly understood, element. The hopes of the modern-day phlogistonians is that we’ll be able to wield the massive amounts of excess renewable energy– for example, wind power overnight, when prices and demand are low, but wind is high- could be used to power electrolysis processes to produce hydrogen.
Cogeneration is another option, as most thermal power plants and fossil fuel extraction operations create a ton of excess heat that is effectively just wasted. Instead of flaring gas, that gas could be burned to support some industrial process. Instead of wasting thermal energy (as happens with roughly 2/3 of thermal power generation energy), it could be combined with a process like hydrogen production. Green? No, not quite. But less dirty? Certainly.
Plenty of folks are working on this question, even though it’s going to take years, and it’s quite possible that we’ll end up discovering a completely different, preferable fuel source! But until then, I’ll remain cautiously optimistic that this is a potential tool in our kit to fight climate change. It’s just not quite there yet.